South Atlantic Connections (SACO)
One critical exchange region for both the departing and returning limbs of the AMOC takes place between the tropical and subtropical South Atlantic gyres (Fig. 1). The departing limb of the AMOC is formed by the southward propagating NADW that crosses eastward the MAR following the major passages at latitudes between 15 and 30ºS, and the returning limb of the AMOC, formed by central and intermediate waters, that follow westwards along the northern margin of the subtropical gyre (Cabré et al 2019; Arumí-Planas et al submitted). These waters come from the Indian Ocean through the warm-salty water route and the Southern Ocean through the cold-fresh water route.
The AMOC and the subtropical gyre
The South Atlantic Subtropical Gyre consists of relatively shallow and salty South Atlantic Central Waters (SACW, in the upper-thermocline) and intermediate waters underneath including SubAntarctic Mode Water (SAMW) and AAIW. These water masses draw a relatively narrow anticyclonic transoceanic gyre, located between about 20°S and 40°S, occupying the middle thermocline (Schmid et al 2000; Schmid and Garzoli 2009) as seen in Figure 1. The boundaries of the gyre consists of the northward Benguela Current (BeC) in the eastern margin (Garzoli and Gordon 1996) and the southward Brazil Current (BC) in the western margin (between 15-20°S and the Brazil-Malvinas Confluence region at 35°S, shrinking with depth) (Goni and Weiner 2001, Rodrigues et al 2007, Goni et al 2011, Orue-Echevarria et al 2019a,b, 2021). In the abyssal layers of the South Atlantic Ocean, the main AABW pathway lies in the western basin with a mass transport that decreases northwards.
Based on observations from the WOCE section at 11ºS carried out in 1994, Schott et al. (2005) found a South Equatorial Current (SEC) transporting 25 Sv northward, binding together the warm-salty and cold-fresh routes of the shallow AMOC. Additionally, the southward deep western boundary southward flow separates between the equator and this latitude (Nof and Olsen 1993, Vilela-Silva et al 2022). Thus, we have chosen 11ºS as a reference section (we will later propose to carry a cruise along this latitude) to assess the intensity of the departing and returning limbs of the AMOC as well as the separation of the returning flow among Deep Western and Eastern Boundary Currents (Figure 1).
As seen in Figure 1, it may appear as if the warm Agulhas rings (ARs) could have a substantial contribution to the northward transport of water mass and heat in the South Atlantic and, therefore, to the AMOC variability. However, when these rings reach the western boundary system, they flow to the south with the Brazil Current (Lutjeharms 2007). Accordingly, rings will contribute to the AMOC only after decaying within the subtropical gyre, what would guarantee that a large fraction of their input to the subtropical gyre gets transferred to the tropical gyre, mainly through the North Brazil Current (NBC) (Lazar et al 2002; Rodrigues et al 2007).
A recent study dealing with the circulation in the South Atlantic Subtropical Gyre found a variable southward BC at 30ºS, with 20.2 ± 0.7 Sv in 2003 and 9.7 ± 0.7 Sv in 2011, overlying the northward AAIW and Upper Circumpolar Deep Water (UCDW) flows (Hernández-Guerra et al 2019). In contrast, the eastern boundary BeC is characterized by high mesoscale eddy activity, east of the Walvis Ridge and before feeding the SEC transporting 15.6 ± 0.9 Sv in 2003 and 11.2 ± 0.8 Sv in 2011. In the ocean interior, the northward flow is mainly located east of the MAR where ARs transport warm and salty water from the Indian to the Atlantic Ocean. Regarding the AABW transport at 30ºS, Hernández-Guerra et al (2019) estimated a northward transport of 6.5 ± 1.9 Sv in 2003 and 8.3 ± 2.1 Sv in 2011. In the northern side of the South Atlantic Subtropical Gyre, Cabré et al (2019) found that the NBC and the North Brazil UnderCurrent (NBUC) carry northward warm and highly saline waters (a total of 38.5 Sv and 2.0 PW), with AMOC subtropical-tropical mass and heat contributions coming from the eastern subtropical boundary (14.9 Sv, 0.64 PW) and the interior subtropical gyre (7.4 Sv, 0.26 PW), which are incremented by (0.2 Sv, 0.20 PW) along its subtropìcal journey (Fig. 3).
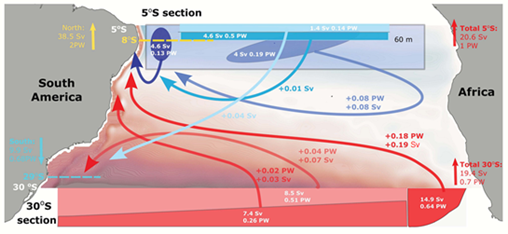
The AMOC and the tropical gyre
The tropical Atlantic Ocean acts as a nexus between the South and North Atlantic basins, connecting southern-origin variability (linked to the Pacific and Indian inflows or Southern Ocean water mass formation) with northern-origin variability (generated in the North Atlantic deep-water formation regions). Surface, central and intermediate waters arrive to the Tropical Atlantic via the westward southern SEC, its northward diversion as the NBUC and NBC, and the equatorial zonal retroflections, i.e. the permanent Equatorial Undercurrent (EUC), including its northern and southern branches, and the seasonal North Equatorial Undercurrent (NECC) (Vallès-Casanova et al 2021).
The southern branch of the SEC carries waters that recirculate in the tropical and subtropical gyres, as well as waters that arrive from the returning limb of the AMOC via two routes: a warm-salty route from the Indian Ocean via the Agulhas Retroreflection and ARs and a cold-fresh route entering from the Pacific through Drake’s passage as the ACC (Casanova et al 2017, Gordon 1986, Rintoul 1991, Rühs et al 2019, Speich et al 2001) (Fig. 1). The SEC feeds into the northward western boundary currents (NBUC and NBC) before reaching the equatorial region, where a substantial fraction of these waters retroflect east (Figs. 3). At deep layers, the DWBC transports NADW to the south and in abyssal layers, the AABW flows northward in the western basin (Stramma and Schott 1999, Frantantoni et al 2000, Rodrigues et al 2007, Hernández-Guerra et al 2019) (Fig. 1).
The returning limb of the AMOC has its deep counterpart in the departing limb, formed by NADW that travels the entire Atlantic from its subpolar Arctic region and the deepest and coldest AABW that originates in the shelfs of the Antarctic continent. All these deep components find their maximum expression and complexity in the South Atlantic. The northward shallow (NBUC and NBC) and also abyssal (AABW) water mass transports have to be compensated with a southward deep transport. During its transit to the Southern Ocean (Figure 2), about 15 Sv of NADW entrain AAIW at its upper boundary and AABW at its lower boundary (de Carvalho Ferreira and Kerr 2017, Johnson 2008, Caínzos et al 2022), increasing the southward mass transport to about 23 Sv near 30°S (Hernández-Guerra et al 2019). When the NADW enters the South Atlantic, a significant fraction of this water mass is intermittently expelled east as relatively narrow deep filaments. These filaments reach the eastern basin through the major fractures in the MAR, particularly the Rio de Janeiro and Rio Grande fracture zones (located at about 25°S and 28°S along 10°W) (Vanicek and Siedler 2002, van Sebille et al 2012, Garzoli et al 2015), flowing below and opposite to the westward intermediate and central waters. As found by Arumí-Planas et al (2022), the eastward flow of NADW lies north of 24ºS (Figure 4). This suggests the convenience of including an additional observational site near 15ºS in order to monitor the entire eastward transport of NADW. It is worthy to remark that one possible consequence of these intermittent filaments is that the southward NADW transport and also its path to the eastern basin changes substantially between years (Hernández-Guerra et al 2019).
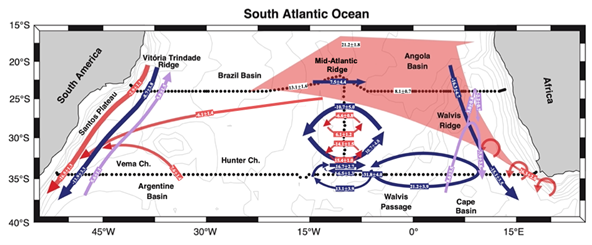
At these deep levels, a freshening of the NADW water mass in the Tropical Atlantic has also been linked to fresher overflows in the North Atlantic in the 1980s to 1990s (e.g. Hummels et al 2015, Fu et al 2018). On the other hand, Heuze (2021) has compiled observational and numerical results to show that AABW penetrates well beyond the equatorial Atlantic, reaching at least 24ºN (Hernández-Guerra et al 2014). Observations confirm that the warming of source AABW has reached the equatorial Atlantic, resulting in a decreased volume transport of its densest component (Herrford et al 2017).
South Atlantic connections: the nexus between the Subtropical Gyre and the Southern Ocean
The Antarctic Circumpolar Current (ACC) flows eastwards around the Antarctic continent, transporting roughly between 100 and 173 Sv (Orsi et al 1995, Cunningham et al 2003, Donohue et al 2016, Olivé Abellò et al 2021, 2023b). Along its path, it connects the Atlantic, Pacific and Indian basins, exchanging heat and freshwater among other properties. Although convergence of net flux estimates has been achieved on basin scales (Ganachaud & Wunsch 2003), the ACC flow into the Atlantic Ocean is critical to establish the magnitude and pathways of the Southern Ocean contribution to the upper branch of the MOC (Fig. 2). Furthermore, the Weddell Sea represents the largest contributor of bottom waters to the deep branch of the MOC in the southern hemisphere (Orsi et al 1999). The study of the connections and exchanges between the Southern Ocean and the subtropical gyre through the abyssal Atlantic Ocean and through the cold-fresh water route from the Drake Passage set the basis of Subproject 2: South Atlantic COnnections: Southern Ocean (SACO-SO).
One key element of the connection between the Southern Ocean and the South Atlantic Ocean is the southward flow of NADW, which is eventually incorporated into the intermediate and shallower layers of the ACC between 50ºS and 60ºS, after upwelling due to the wind-induced divergence (Purich et al 2016). After further modification along its interoceanic travel via intense air-sea interaction, a fraction of these upwelled waters will finally return to the South Atlantic in the form of the cold-fresh intermediate Pacific waters and the warm-salty Indian central waters. These connections take place in the Atlantic sector of the Southern Ocean through two main routes: the ACC transports through the Drake Passage and the flow of Indian waters and Agulhas rings and filaments through the southern tip of Africa. They conform the cold-fresh and the warm-salty water routes, respectively. Early estimates suggest that the cold-fresh water route represents a relatively minor contribution as compared with the Indian Ocean inflow (Sloyan and Rintoul 2001). However, two recent studies of the ACC through the Drake Passage show transports substantially greater than previously calculated (Olivé Abelló et al 2021, 2022b), and these transports may be increasing as a result of anthropogenic climate change (Shi et al 2021).
One key element of the connection between the Southern Ocean and the South Atlantic Ocean is the southward flow of NADW, which is eventually incorporated into the intermediate and shallower layers of the ACC between 50ºS and 60ºS, after upwelling due to the wind-induced divergence (Purich et al 2016). After further modification along its interoceanic travel via intense air-sea interaction, a fraction of these upwelled waters will finally return to the South Atlantic in the form of the cold-fresh intermediate Pacific waters and the warm-salty Indian central waters. These connections take place in the Atlantic sector of the Southern Ocean through two main routes: the ACC transports through the Drake Passage and the flow of Indian waters and Agulhas rings and filaments through the southern tip of Africa. They conform the cold-fresh and the warm-salty water routes, respectively. Early estimates suggest that the cold-fresh water route represents a relatively minor contribution as compared with the Indian Ocean inflow (Sloyan and Rintoul 2001). However, two recent studies of the ACC through the Drake Passage show transports substantially greater than previously calculated (Olivé Abelló et al 2021, 2022b), and these transports may be increasing as a result of anthropogenic climate change (Shi et al 2021).
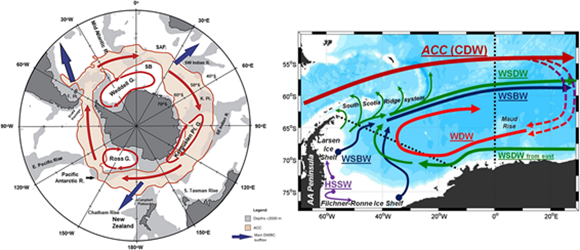
The dense precursors of AABW are formed through intense air-sea-ice interactions in selected locations of the Antarctic continental platform, mainly in the southern and western Weddell and Ross Seas, (e.g. Fahrbach et al 1995). These dense precursors will eventually cascade down the continental slope (Fig. 7), mixing with WDW, and thus being transformed into Weddell Sea Bottom Water (WSBW) and Weddell Sea Deep Water (WSDW) (e.g. Fahrbach et al., 2001). Subsequently, both WSDW and WSBW will circulate along the western boundary of the Weddell Gyre and, after further mixing, more WSBW will be converted into WSDW, which is less dense and can cross the South Scotia Ridge through specific passages (Meredith et al 2011). Consequently, the South Scotia Ridge is a key location for the final transformation of WSDW and its export to the South Atlantic abyss in the form of AABW. Novel observations from the Orkney Pasage suggest that this transformation takes place through bottom-boundary mixing via submesoscale dynamical instabilities and possibly enhanced by internal tides (Naveira Garabato et al 2019). The export of modified WSDW (i.e. AABW) through the Orkney Passage has been well documented by British scientists (e.g. Abrahamsen et al., 2019). However, the plausible AABW export through the passages located further west of the Orkney plateau, and the processes involved, have received comparatively much less attention and need to be yet well documented.
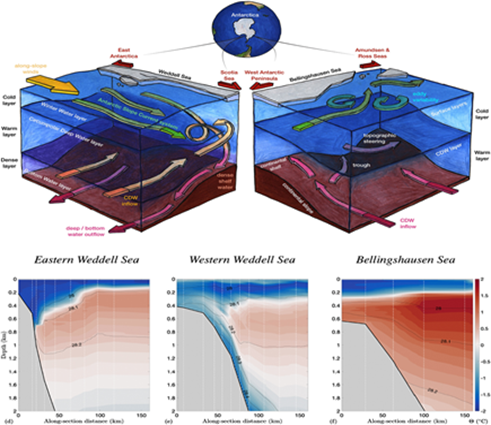
Remarkably, the export of bottom shelf waters in the southern and western Weddell Sea enhances the onshore transport of the relatively warm MCDW (i.e. WDW). This is a very relevant process, as the MCDW (originated from NADW) is experiencing substantial anthropogenic warming (Schmidtko et al 2014) and, once it reaches the vicinity of the Antarctic ice shelves, it can drive intense ice-shelf basal melting and thus increase future sea level rise (Hellmer et al. 2012, Rignot et al 2021). Eddy-induced onshore transport of MCDW concentrates in intermediate layers away from boundary layers (Thompson et al 2014). In addition to that, the mixing induced by submesoscale motions and internal tides, together with the wind-driven upwelling taking place within the Weddell Gyre, may also favour the intrusions of MCDW into the Antarctic shelf. These processes also influence the heat and salt content of the WSDW and WSBW formed after the downslope export of the dense precursors of AABW (Ruan et al 2017, Llanillo et al., 2023).
The observation of these oceanographic processes requires high-resolution spatiotemporal sampling, which may be accomplished via instrumented gliders (Fig. 8) and moorings. Consequently, the deployment of such scientific instruments is a key step in the SACO project.
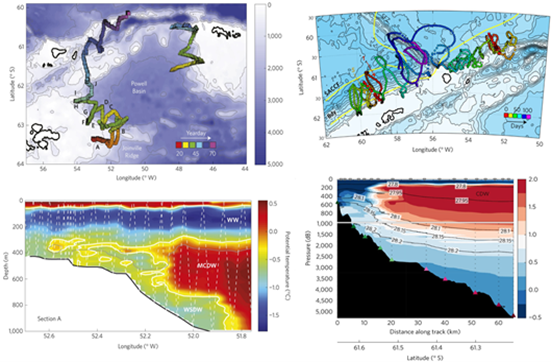